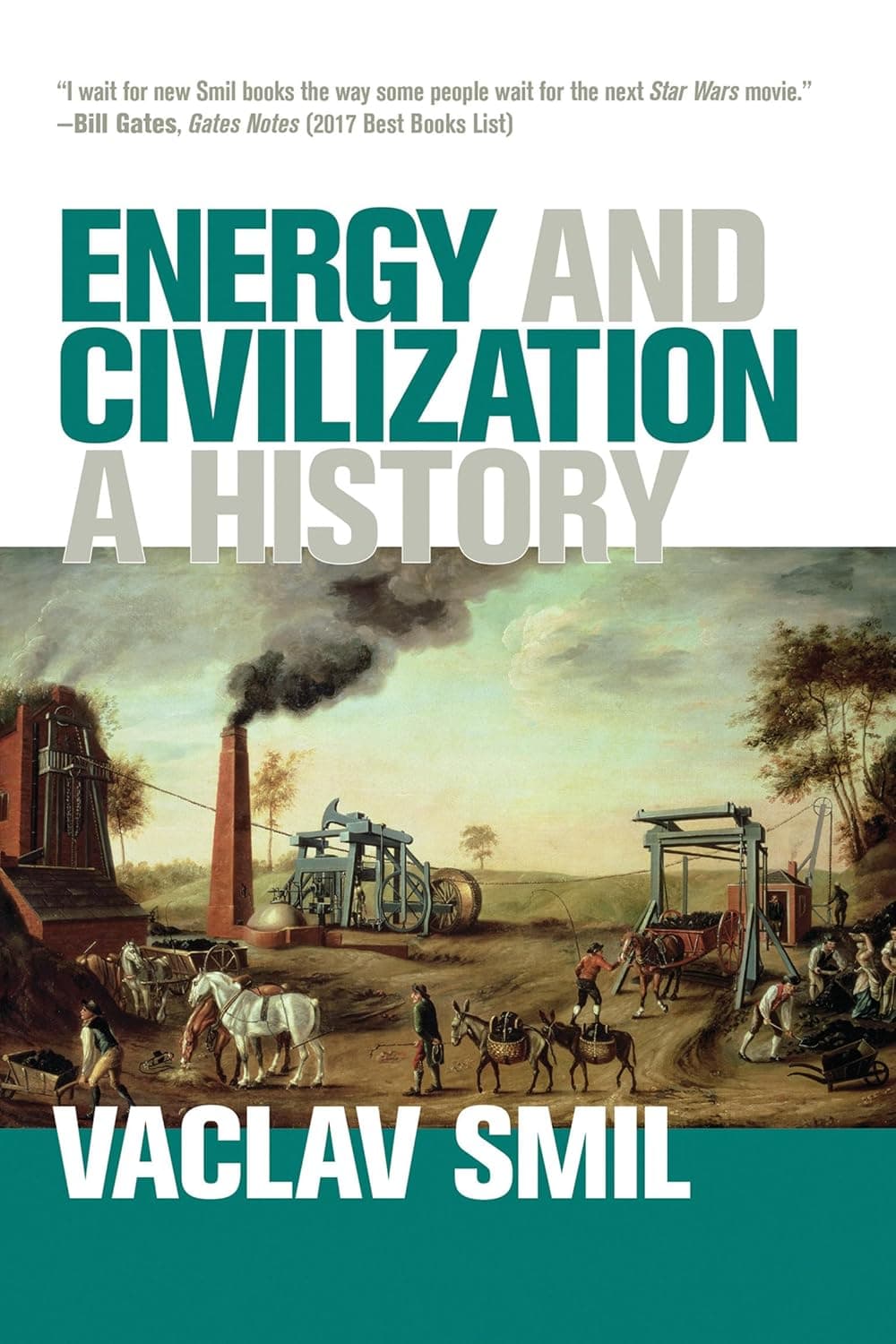
Energy and Civilization
By: Vaclav Smil
Category: History
Finished:
Highlights
1: Energy and Society
Energy is the only universal currency: one of its many forms must be transformed to get anything done.
From a fundamental biophysical perspective, both prehistoric human evolution and the course of history can be seen as the quest for controlling greater stores and flows of more concentrated and more versatile forms of energy and converting them, in more affordable ways at lower costs and with higher efficiencies, into heat, light, and motion.
“Other things being equal, the degree of cultural development varies directly as the amount of energy per capita per year harnessed and put to work ”
Only a tiny part of the incoming radiant energy, less than 0.05%, is transformed by photosynthesis into new stores of chemical energy in plants, providing the irreplaceable foundation for all higher life.
Every form of energy can be turned into heat, or thermal energy. No energy is ever lost in any of these conversions. Conservation of energy, the first law of thermodynamics, is one of the most fundamental universal realities. But as we move along conversion chains, the potential for useful work steadily diminishes (box 1.2). This inexorable reality defines the second law of thermodynamics, and entropy is the measure associated with this loss of useful energy. While the energy content of the universe is constant, conversions of energies increase its entropy (decrease its utility).
This unidirectional entropic dissipation leads to a loss of complexity and to greater disorder and homogeneity in any closed system. But all living organisms, whether the smallest bacteria or a global civilization, temporarily defy this trend by importing and metabolizing energy. This means that every living organism must be an open system, maintaining a continuous inflow and outflow of energy and matter.
One calorie is the amount of heat needed to raise the temperature of 1 cm3 of water by 1°C.
Today’s standard unit of power, one watt, is equal to the flow of one joule per second.
One horsepower is equal to about 750 watts.
even where abundant, low-energy-density foods could never become staples.
Power density is the rate at which energies are produced or consumed per unit of area and hence is a critical structural determinant of energy systems
The power density of sustainable annual tree growth in temperate climates is at best equal to 2% of the power density of energy consumption for traditional urban heating, cooking, and manufactures. Consequently, cities had to draw on nearby areas at least 30 times their size for fuel supply.
Yet another rate, one that has assumed great importance with advancing industrialization, is the efficiency of energy conversions. This ratio of output/input describes the performance of energy converters, be they stoves, engines, or lights. While we cannot do anything about the entropic dissipation, we can improve the efficiency of conversions by lowering the amount of energy required to perform specific tasks
Candles convert just 0.01% of chemical energy in tallow or wax to light. Edison’s light bulbs of the 1880s were roughly ten times as efficient.
fluorescent lights have efficiencies up to 15%, as do light-emitting diodes (USDOE 2013). This means that about 9% of energy in natural gas ends up as light, a 90-fold gain since the late 1880s.
When efficiencies are calculated for the production of foodstuffs (energy in food/energy in inputs to grow it), fuels, or electricity they are usually called energy returns.
An abundance of high-energy-density coal ideal for fueling steam engines (the often used adjective “smokeless” must be seen in relative terms) was clearly a major factor contributing to the British dominance of nineteenth-century maritime transport, as neither France nor Germany had large coal resources of comparable quality.
2: Energy in Prehistory
Human walking costs about 75% less energy than both quadrupedal and bipedal walking in chimpanzees.
The brain’s specific energy need is roughly 16 times that of skeletal muscles, and the human brain claims 20–25% of resting metabolic energy , compared to 8–10% in other primates and just 3–5% in other mammals
Fish and Lockwood (2003), Leonard, Snodgrass, and Robertson (2007), and Hublin and Richards (2009) confirmed that diet quality and brain mass have a significantly positive correlation in primates, and better hominin diets, including meat, supported larger brains, whose high energy need was partly offset by a reduced gastrointestinal tract
While extant nonhuman primates have more than 45% of their gut mass in the colon and only 14–29% in the small intestine, in humans those shares are reversed, with more than 56% in the small intestine and only 17–25% in the colon, a clear indication of adaptation to high-quality, energy-dense foods (meat, nuts) that can be digested in the small intestine.
Increased meat consumption also helps to explain human gains in body mass and height, as well as smaller jaws and teeth.
Whereas allometric equations predict about five 50 kg mammals/km2, chimpanzee densities are between 1.3 and 2.4 animals/km2, and the densities of hunter-gatherers surviving into the twentieth century were well below one person/km2 in warm climates, only 0.24 in the Old World and 0.4 in the New World
Mollusk collecting, fishing, and near-shore hunting of sea mammals sustained the highest foraging densities and led to semipermanent, even permanent, settlements. The coastal villages of the Pacific Northwest, with their large houses and organized communal hunting of sea mammals, were exceptional in their sedentism.
Generally less than 30% of the ingested phytomass is digested; most of it is respired, and in mammals and birds only 1–2% of it is converted into zoomass. As a result, the most commonly hunted herbivores embodied less than 1% of the energy initially stored in the phytomass of the ecosystems they inhabited. This reality explains why hunters preferred to kill animals that combined a relatively large adult body mass with high productivity and high territorial density: wild pigs (90 kg) and deer and antelopes (mostly 25–500 kg) were common targets.
In light of the unimpressive physical endowment of early humans and the absence of effective weapons, it is most likely that our ancestors were initially much better scavengers than hunters
Large predators—lions, leopards, saber-toothed cats—often left behind partially eaten herbivore carcasses. This meat, or at least the nutritious bone marrow, could be reached by alert early humans before it was devoured by vultures, hyenas, and other scavengers.
human bipedalism and ability to sweat better than any other mammal made it also possible to chase to exhaustion even the fastest herbivores
Horses lose water at an hourly rate of 100 g/m2 of their skin, and camels lose up to 250 g/m2, but people lose more than 500 g/m2, with peak rates of more than 2 kg/hour
People can also drink less than they perspire, and make up for any temporary partial dehydration hours later. Running turned humans into diurnal, high-temperature predators that could chase animals to exhaustion
Hunters running barefoot reduced their energy costs by about 4% (and had fewer acute ankle and chronic lower leg injuries) than modern runners with athletic shoes.
Late Pleistocene hunters may have become so skillful that many students of the Quaternary era concluded that hunting was largely (even completely) responsible for a relatively rapid disappearance of the late Paleolithic megafauna, animals with a body mass greater than 50 kg
All wild meat is an excellent source of protein, but most of it contains very little fat, and hence it has very low energy density—less than half that of grains for small, lean mammals. Not surprisingly, there was a widespread hunting preference for large and relatively fatty species.
Thanks to its high fat content (about 15%), salmon has an energy density (9.1 MJ/kg) nearly three times that of cod.
The high energy density of blubber (about 36 MJ/kg) and muktuk (skin and blubber, which also has a vitamin C content comparable to that of grapefruit) resulted in a more than 2,000-fold energy gain in hunting.
A food supply dependent on a few seasonal energy flows required extensive, and often elaborate, storage. Storage practices included caching in permafrost; drying and smoking of seafood, berries, and meats; storing of seeds and roots; preservation in oil; and the making of sausages, nut-meal cakes, and flours. Large-scale, long-term food storage changed foragers’ attitudes toward time, work, and nature and helped stabilize populations at higher densities
contrary to all popular claims about the benefits of Paleolithic diets, we still cannot reconstruct the representative composition of pre-agricultural subsistence.
3: Traditional Farming
Tools and simple machines made field operations easier (thus providing a mechanical advantage) and faster, raised productivity, and enabled fewer people to grow more food, and the resulting energy surplus could be invested in structures and actions: without sickle and plow there would be no cathedrals—or no European voyages of discovery.
Although seed drills were used in Mesopotamia as early as 1300 BCE, and sowing plows were used by the Han Chinese, broadcast seeding by hand—wasteful and resulting in uneven germination—remained common in Europe until the nineteenth century.
genetic mutations increased starch digestion in dogs relative to the carnivorous diet of wolves , a crucial step in domesticating the species
All animal foods and all mushrooms supply perfect proteins (with adequate proportions of all nine essential amino acids), but all four leading staple grains (wheat, rice, corn, millet) and other important cereals (barley, oats, rye) are deficient in lysine, while tubers and most legumes are short of methionine and cysteine.
No quest for higher yields could succeed without three essential advances.
- The first one was a partial replacement of human work by animal labor.
- Second, irrigation and fertilization moderated , if not altogether removed, the two key constraints on crop productivity, shortages of water and nutrients.
- Third, growing a greater variety of crops , either by multicropping or in rotations, made traditional cultivation both more resilient and more productive.
Unlike cattle, whose body mass is almost equally divided between the front and the rear, horses’ fronts are notably heavier than their rears (ratio of about 3:2), and so the pulling animal can take a better advantage of inertial motion than cattle
a horse’s leg anatomy gives the animal a unique advantage by virtually eliminating the energy costs of standing. The horse has a very powerful suspensory ligament running down the back of the cannon bone and a pair of tendons (superficial and deep digital flexors) that can “lock” the limb without engaging muscles.
The water demand of crops depends on many environmental, agronomic, and genetic variables, but the total seasonal need is commonly about 1,000 times the mass of the harvested grain. Up to 1,500t of water are needed to grow 1t of wheat, and at least 900 t must be supplied for every tonne of rice. About 600 t will suffice for a tonne of corn, a more water-efficient C4 crop and the staple grain with the highest water use efficiency
The organic fertilizer with the highest nitrogen content (around 15% for the best deposits) is guano , droppings of seabirds preserved in the dry climate of islands along the Peruvian coast.
Cultural differences aside, nearly all traditional peasants behaved as gamblers. They tried to stay on the slim margins of food surplus too long, betting that the weather would help to produce another fair harvest next year. But given the low staple grain yields and relatively high seed/harvest ratios they lost repeatedly, and often catastrophically.
The energy cost of pregnancy and of bringing up another child is negligible compared to its labor contribution, which can start at a very early age.
4: Preindustrial Prime Movers and Fuels
Two principal roads led to higher outputs and better efficiencies. The first one was multiplication of small forces, primarily a matter of superior organization, especially with the application of animate energies. The second one was technical innovation, which introduced new energy conversions or increased the efficiencies of established processes.
For example, monumental structures built by virtually every old high culture demanded both massed labor and extensive applications of labor-easing devices, starting with simple levers and inclined planes and eventually including pulleys, cranes, windlasses, and treadwheels.
Prime Movers
But for millennia the only pale imitation was to attach incendiary materials to arrowheads, or to hurl them in containers from catapults. Sulfur, petroleum, asphalt, and quicklime were used in these incendiary mixtures. Only the invention of gunpowder combined the propulsive force with great explosive and inflammatory power.
Animate Power
There were only two practical ways in which the delivery of useful animate power could be increased: either by concentrating individual inputs or by using mechanical devices to redirect and amplify muscular exertions.
The three simplest aids providing mechanical advantage—levers, inclined planes, and pulleys—were used by virtually all old high cultures
Naturally, the same amount of work can be accomplished by applying a greater force over a shorter distance or a smaller force over a longer distance: any device that converts a small input force into a larger output force provides a mechanical advantage whose magnitude is measured simply as a dimensionless ratio of the two forces.
A worker raising building materials with Archimedean potential pulley (D) could lift (ignoring friction) a 200 kg stone with a force of only 25 kg, but a lift of 10 m will require pulling 80 m of the counterweight cord. A ratchet and pawl can be used to interrupt this effort anytime.
Water Power
But my estimates show that even with very liberal assumptions regarding the unit power and adoption of waterwheels throughout the Roman Empire, water power contributed only a fraction of 1% of the useful mechanical energy supplied by people and draft animals
Human and animal muscles could never convert energy at such high, concentrated, continuous, and reliable rates—but only such deliveries could increase the scale, speed, and quality of countless food-processing and industrial tasks. Yet it took a long time for typical waterwheels to reach capacities surpassing the power of large harnessed animal teams.
Even when assuming that flour supplied half of an average person’s daily food energy intake, a small water mill, manned by fewer than 10 workers, would grind enough in a day (10 hours of milling) to feed some 3,500 people, a fair-sized medieval town, while hand milling would have required at least 250 laborers.
Waterwheels were the most efficient traditional energy converters. Their efficiencies were superior even when compared to the best steam engines, whose operation converted less than 2% of coal into useful power by 1780, and usually no more than 15% even by the end of the nineteenth century (Smil 2005).
By 1880 large-scale coal mining and more efficient engines had made steam cheaper than water power virtually anywhere in the United States. Before the end of the nineteenth century most water turbines had stopped delivering direct power and had started to turn electricity generators instead.
Wind Power
As in the case of waterwheels, the contribution of windmills as providers of stationary power peaked during the nineteenth century. In the UK their total reached 10,000 in 1800; during the late nineteenth century 12,000 worked in the Netherlands while18,000 worked in Germany; and by 1900 about 30,000 mills (with a total capacity of 100 MW) were installed in countries around the North Sea
In the United States, several million units were erected between 1860 and 1900 during the country’s westward expansion, and their numbers began to decline only in the early 1920s.
Biomass Fuels
The abundance or scarcity of fuel affected house design, as well as dressing and cooking practices. Provision of these energies was one of the principal reasons for traditional deforestation.
Wood and Charcoal
Freshly cut mature hardwoods (leafy trees) are typically 30% water, while softwoods (conifers) are well over 40%. Such wood burns inefficiently as a significant part of the released heat goes into vaporizing released moisture rather than heating a cooking pot or a room. When wood has more than 67% of moisture it will not ignite.
Cut wood was stacked, sheltered, and let dry for at least a few months, but even in dry climates it still retained about 15% moisture. In contrast, charcoal contains only a trace of moisture , and it was a biomass fuel always preferred by those who could afford its price.
Crop Residues and Dung
The low density of crop residues also meant that open fires and simple stoves had to be stoked almost constantly. Because of a number of competitive nonenergy uses, crop residues were often in short supply. Legume residues were an excellent high-protein feed and fertilizer. Cereal straw makes a good ruminant food and animal bedding; many societies (including England and Japan) used it for thatching house roofs, and it was also a raw material for manufacturing simple tools and domestic articles.
A little-appreciated reality is dung’s essential contribution to America’s westward expansion
Food Preparation
In light of the dominance of cereals in the nutrition of all high cultures, milling of grains was certainly the most important food-processing need in history.
Milling produces flours of various fineness that could be used for the preparation of highly digestible foods, above all breads and noodles.
A donkey-powered mill (energy input at the rate of 300 W) produced from less than 10 kg/h to 25 kg/h (Forbes 1965), while millstones driven by a small waterwheel (1.5 kW) would grind flour at rates between 80 and 100 kg/h.
Flour would have been used to bake bread supplying at least half of all food energy in average dietary intake (but bread’s share was often more than 70%).
Consequently, a single mill would have produced enough flour in a 10-hour shift to feed 2,500–3,000 people , a fair-sized medieval town.
Heat and Light
In heating, the much-needed transition from wasteful, unregulated open fires to more efficient arrangements was very slow. Merely moving an open fire into a three-sided fireplace brought only a marginal efficiency gain. Well-stoked fireplaces could keep an unattended fire overnight, but their heating efficiencies were poor. The best rates were close to 10%, but more typical performances were just around 5%. And often a working fireplace, warming its immediate vicinity with radiated heat but drawing the warm inside air outside, was actually causing an overall heat loss in the room. When this draft was impeded the combustion could produce dangerous, even lethal, levels of carbon monoxide.
Transportation and Construction
Moving on Land
Arellano and Kram (2014) showed that body weight support and forward propulsion account for about 80% of the total cost of running; leg swinging claims about 7%, and maintaining lateral balance about 2%—but arm swinging cuts the overall cost by about 3%.
Experienced riders on a fit animal had no difficulty riding 50–60 km/day, and by changing horses they could cover more than 100 km/day in emergencies.
Pulling a 140 t stone, the heaviest block at Ollantaytambo in southern Peru, up the ramp required the coordinated force of about 2,400 men
How were more than 2,000 men harnessed to pull in concert? How were they arranged to fit into the confines of narrow (6–8 m) Inca ramps? And how did the people in ancient Brittany handle the Grand Menhir Brise (Niel 1961), at 340 t the largest stone erected by a European megalithic society?
Curiously, it was also only during that time that European and American mechanics came up with a practical version of the most efficient human-powered locomotive vehicle, the modern bicycle. For generations bicycles were clumsy, even dangerous, contrivances that had no chance of mass adoption as vehicles of convenient personal transport. Rapid improvements came only during the 1880s.
Until the early 1980s there were no private cars in China, and until the late 1990s most commuters rode bicycles even in the country’s large cities.
Oared Ships and Sail Ships
The maximum capabilities for sailing near the wind have advanced by more than 100° since the beginning of sailing. Early Egyptian square-sailed ships could manage only a 150° angle, while medieval square rigs could proceed slowly with the wind on their beam (90°), and their post-Renaissance successors could move at an angle of just about 80° into the wind. Only the use of asymmetrical sails mounted more in line with the ship’s long axis and capable of swiveling around their masts made sailing closer to the wind possible.
Ships combining square sails with triangular mizzens could manage 60°, and fore-and-aft rigs (including triangular, lug, sprit and gaff sails) could come as close as 45° to the wind. Modern yachts come very close to 30°, the aerodynamic maximum.
Roman ships pushed by the northwesterlies could make the Messina-Alexandria run in just 6–8 days, but the return could take 40–70 days.
In 1492 Columbus’s Santa Maria had a capacity of 165 t, and the Trinidad, Magellan’s ship, had a mere 85 t. A century later vessels of the Spanish Great Armada (sailing in 1599) averaged 515 t.
By 1800 British ships in the Indian fleet had capacities of about 1,200 t.
Buildings and Structures
The enormous variety of building styles and ornaments can be reduced to only four fundamental structural members: walls, columns, beams, and arches.
In contrast to our conjectures about the construction of the largest pyramids, there is little mystery about the way such classical structures as the Parthenon or the Pantheon were built
Metallurgy
Nonferrous Metals
The first uses of brass date to the first century BCE. The alloy became widely used in Europe only during the eleventh century, and became common only after 1500.
Iron and Steel
Small iron objects were produced in Mesopotamia during the first half of the third millennium BCE, but ornaments and ceremonial weapons became more common only after 1900 BCE. The extensive use of iron dates only to after 1400 BCE, and the metal became truly abundant after 1000 BCE.
African iron making is also ancient, but the metal was never smelted by any New World society.
Iron melts at 1535°C; an unaided charcoal fire can reach 900°C, but a forced air supply can raise its temperature close to 2000
In 1720, 60 British furnaces produced about 17,000 t of pig iron, requiring, with 40 kg of wood per kilogram of metal, about 680,000 t of wood. Forging the metal to produce 12,000 t of bars added, with 2.5 kg of charcoal per kilogram of bars, another 150,000 t, for a total annual consumption of some 830,000 t of charcoaling wood. With an average productivity of 7.5 t/ha, this would have required about 1,100 km2 of forests and coppiced growth for sustainable harvests.
Even when assuming a high average increment of 7 t/ha in secondary-growth forests, a sustainable supply of that wood would have required annual harvests from nearly 180,000 km2 of forest, an area equal to Missouri (or a third of France), represented by a square whose side would reach from Philadelphia to Boston or from Paris to Frankfurt. Obviously, even a forest-rich America could not afford to energize its iron ore smelting with charcoal.
The spreading availability of iron and steel gradually led to a number of profound social changes. Iron saws, axes, hammers, and nails sped up house construction and improved its quality. Iron kitchenware and a variety of other utensils and objects, ranging from rings to rakes, from grates to graters, made it easier to cook and run a household. Iron horseshoes and plowshares were instrumental in advancing the intensification of cropping. On the destructive side, warfare was revolutionized first by flexible chain-mail suits, helmets, and heavy swords, later by guns, iron cannonballs, and more reliable firearms. These trends were greatly accelerated with the introduction of coke-based iron smelting and the emergence of the steam engine.
Warfare
The explosive reaction of chemicals could propel projectiles faster and farther and increase their destructive impact. For centuries this impact was limited by the clumsy designs of personal weapons (front- and breach-loading rifles), but gunpowder gained ever greater importance as the propellant of cannonballs.
All prehistoric land warfare and all conflicts of ancient and early medieval eras were powered solely by human and animal muscles. Warriors wielded daggers, axes, and swords in close combat, on foot or on horseback. They used spears and lances, and they drew bows and much more powerful crossbows (both the Chinese and the Greeks used them since the fourth century BCE) to shoot arrows whose impact would injure and kill unprotected enemies as far as 100–200 m away.
Animate Energies
These simple pieces of metal providing footholds for a rider were first used in China in the early third century CE and then diffused westward; they gave riders unprecedented support and stability in the saddle (Dien 2000). Without them a fighter clad in armor could not even mount a larger (and sometimes also partially armored) horse, and would have been unable to fight effectively with a lance or a heavy sword while on its back.
5: Fossil Fuels, Primary Electricity, and Renewables
The transition to fossil fuels has also entailed two classes of fundamental qualitative improvements, and only their accumulation and combination have produced the energetic foundations of the modern world.
The first category of these advances was the invention, development, and eventually mass-scale diffusion of new ways to convert fossil fuels : by introducing new prime movers—starting with steam engines and progressing to internal combustion engines, steam turbines, and gas turbines—and by coming up with new processes to transform raw fuels, including the production of metallurgical coke from coal, the refining of crude oils to produce a wide range of liquids and nonfuel materials, and the use of coals and hydrocarbons as feedstock in new chemical syntheses.
The second class of inventions used fossil fuels to produce electricity, an entirely new kind of commercial energy. Any solid, liquid, or gaseous fuel could be burned, its released heat used to convert water into steam, and the steam used to rotate turbogenerators and produce electricity.
The long-term trend is clear: we have been converting a steadily rising share of fossil fuels to thermal electricity, and we have also been expanding the capacities for primary electricity generation because electricity is the most convenient, most versatile, and, at the point of its use, the cleanest form of modern energy.
The Great Transition
Steam engine became the leading inanimate prime mover of the nineteenth century. Internal combustion engines and steam turbines started to make commercial inroads during the 1890s. Before 1950 gasoline and diesel engines became the dominant prime movers in transportation and steam turbines in the large-scale generation of electricity (Smil 2005); the widespread use of gas turbines (stationary for electricity generation or powering jetliners and ships) came only after 1960
The Beginnings and Diffusion of Coal Extraction
Every transition to a new form of energy supply has to be powered by the intensive deployment of existing energies and prime movers : the transition from wood to coal had to be energized by human muscles, coal combustion powered the development of oil, and, as I stress in the last chapter, today’s solar photovoltaic cells and wind turbines are embodiments of fossil energies required to smelt the requisite metals, synthesize the needed plastics, and process other materials requiring high energy inputs.
The latest leading economy to accomplish the transition from phytomass to coal was China , where the process was delayed by the endless twentieth-century crises: they started with the collapse of the imperial rule in 1911, continued with the protracted civil war between the Communists and the Kuomintang (1927–1936, 1945–1950) and the war with Japan (1933–1945), and afterward came decades of Maoist economic mismanagement, including the world’s largest, Mao-made, Great Famine (1958–1961) and the insanely named Cultural Revolution (1966–1976). As a result, it was only in 1965 that biomass fuels began to supply less than half of China’s primary energy; by 1983 their share had fallen below 25% and by 2006 below 10%
From Charcoal to Coke
Steam Engines
At the same time, commercialization and the widespread adoption of steam engines advanced slowly, taking more than a century, and even during the years of their rapid diffusion, after 1820, they had to compete (as already noted in chapter 4) with waterwheels and turbines.
A profusion of new designs brought more efficient and faster machines. By 1900 the best locomotive engines operated at pressures up to five times higher than in the 1830s, and with efficiencies of more than 12% (Dalby 1920). Speeds over 100 km/h became common, and during the 1930s streamlined locomotives approached, and even surpassed, 200 km/h
The cumulative technical progress was remarkable: the largest machines designed during the 1890s were about 30 times more powerful than those in 1800 (3 MW vs. about 100 kW), and the efficiency of the best units was ten times better, 25% versus 2.5% (fig. 5.5). This huge performance gain, translating into large fuel savings and less air pollution, came mainly from a hundredfold rise in operating pressures, from 14 kPa to 1.4 MPa.
But steam engines became victims of their own success: as their typical efficiencies rose and their highest capacities reached unprecedented levels (orders of magnitude above any traditional prime mover), they began to encounter their inherent limitations as more was demanded of them than they could deliver (Smil 2005). Even after more than a century of improvements the most commonly used steam engines remained highly inefficient: by 1900 the typical steam locomotive wasted 92% of coal fed into its boiler. And they remained heavy, limiting mobile uses beyond water and rails where it was easier to support their mass
Oil and Internal Combustion Engines
In North America oil was collected from natural seeps in western Pennsylvania during the late eighteenth century and sold as a medicinal “Seneca oil,” and in France oil sands were exploited since 1745 in Alsace, near Merkwiller-Pechelbronn, where the first small refinery was built in 1857
But there was only one place in the preindustrial world with a long history of collecting crude oil, Baku’s Absheron peninsula on the Caspian Sea in today’s Azerbaijan.
The world’s first commercial oil-distilling factory was built by the Russians in 1837 in Balakhani, and in 1846 they sank the world’s first (21 m deep) exploratory oil well in Bibi-Heybat and thus began the exploitation of one of the world’s giant oil fields, still producing today.
The American search for oil was motivated by finding a replacement of expensive whale oil rendered on board ships from the blubber of sperm whales and burned in lamps (Brantly 1971). Americans had the world’s largest whaling fleet—the total peaked in 1846 at more than 700 vessels—and during the early 1840s they brought about 160,000 barrels of sperm oil to New England’s ports every year (Starbuck 1878; Francis 1990).
But the first North American well was dug manually in Canada in 1858, by Charles Tripp and James Miller Williams near Black Creek in Lambton County in southwestern Ontario, bringing the world’s first oil boom and the renaming of the hamlet as Oil Springs
Anthony Francis Lukas made a spectacular discovery of the Spindletop field near Beaumont in southern Texas with a gusher that produced 100,000 barrels/day on January 10, 1901
Most of these discoveries were hydrocarbon fields containing both crude oil and associated natural gas, but gas was rarely used during the early decades of the hydrocarbon industry because without compressors and steel pipes it could not be moved over long distances, and it was simply vented.
In contrast, the high energy density of liquid fuels refined from crude oil (gasoline, kerosene, and fuel oils) and their easy portability made them the superior energy source for transportation , and the invention and rapid adoption of the internal combustion engine opened up a huge new market for their use.
When the oil extraction began to expand during the 1860s there were no commercial internal combustion engines capable of powering vehicles, but again within about 25 years, two German engineers had built their first practical automotive engines and created a new fuel demand that has yet to see its global peak more than 130 years later.
Gasoline has an energy density of 33 MJ/L (about 1,600 times that of the town gas used by Otto) and a very low flashpoint (−40°C), which makes engines easy to start.
In November 1885 they used a subsequent air-cooled version to power the world’s first motorcycle, and in March 1886 their larger (0.462 L, 820 W), 600 rpm, water-cooled design was mounted on a wooden-wheeled coach
Electricity
The high operating cost and limited battery capacity made small DC motors inferior prime movers compared to steam engines.
Technical Innovations
But by 1900 many people in major Western cities lived in societies whose technical parameters were almost entirely different from those that dominated the world in 1800 and that were, in their fundamental features, much closer to our lives in the year 2000.
in 1800 the world consumed about 20 EJ of energy (an equivalent of less than 500 Mt of crude oil), of which 98% was phytomass, mostly wood and charcoal; by 1900 the total primary energy supply had more than doubled (to about 43 EJ, equal to just over 1 Gt of crude oil), and half of it came from fossil fuels, mostly from coal.
In 1800 the most powerful inanimate prime mover, Watt’s improved steam engine, had a capacity just above 100 kW; in 1900 the largest steam engines rated 3 MW, or 30 times as much.
First, the global expansion of coal mining and hydrocarbon production raised the annual extraction of fossil carbon roughly 20-fold between 1900 and 2015: from 500 Mt in 1900 to 6.7 Gt a century later and to about 9.7 Gt in 2015
Third, there has been a clear secular shift toward higher-quality fuels , that is, from coals to crude oil and natural gas, a process that has resulted in relative decarbonization (a rising H:C ratio) of global fossil fuel extraction, while absolute levels of CO2 emitted to the atmosphere have been rising except for a few temporary slight annual declines.
Coals
Coal combustion launched the thermal electricity generation during the 1880s and in every traditional coal-mining country, and that dependence only grew as large central power plants were constructed after World War II, when the increasing share of surface extraction made coal even more affordable.
The U.S. coal output was surpassed by the Chinese production in 1985, and coal has been by far the most important energizer of China’s extraordinary economic growth
Because the fuel generates more CO2 per unit of released energy than any other fossil fuel—the rates are typically more than 30 kg C/GJ for coal, about 20 kg C/GJ for liquid hydrocarbons, and less than 15 kg C/GJ for natural gas—its future in a world concerned about rapid global warming is uncertain.
Hydrocarbons
The first new large market was created by the introduction of affordable automobiles, starting before World War I with Ford’s Model T, and by the rapid post–World War II rise in car ownership; the second one began with the introduction of jet engines in commercial aviation during the 1950s, an innovation that changed flying from a very expensive and a rare experience to a mass global industry
And the most recent production advance is the rising extraction from nonconventional sources of crude oil, including heavy oils (in many places around the world), oil embedded in tar sands (Alberta, Venezuela), and extraction by hydraulic fracturing to produce oil from shales.
This technique, pioneered in the United States, has proved so successful that America became once again the world’s largest producer of crude oil and other petroleum liquids
All of this has brought four notable outcomes. First, global oil production grew roughly 200-fold during the twentieth century ; by 2015 it was (at over 4.3 Gt) about 20% higher than in the year 2000, and since 1964, when its energy content surpassed that of coal extraction, it has been the world’s mostly commonly used fuel.
Electricity
Nuclear fission’s arrival as the other major way to raise steam for thermal electricity generation was accelerated by World War II. The first demonstration of the phenomenon, by Lise Meitner and Otto Frisch in December 1938, was followed by the first sustained chain reaction, at the University of Chicago on December 2, 1942. The first nuclear bomb was tested in July 1945, and two bombs were dropped three days apart in August 1945
the first major postwar U.S. nuclear program was to develop nuclear reactors for the propulsion of submarines : the Nautilus was launched in January 1955, and almost immediately Hyman Rickover (1900–1986), leader of the nuclear submarine program, was put in charge of reconfiguring the reactor for commercial electricity generation (Polmar and Allen 1982). The first U.S. nuclear power station, Shippingport, in Pennsylvania, began operating in December 1957, more than a year after the British Calder Hall was started, in October 1956.
Prime Movers in Transportation
Reciprocating aircraft engines improved very rapidly. Those powering Boeing’s 1936 Clipper (a large hydroplane flying scheduled service between the U.S. West Coast and East Asia) were about 130 times more powerful than Wright’s 1903 machine , whose weight/power ratio was more than ten times as high
In 1952 the British Comet became the first passenger jet, but structural defects rather than engine problems led to three fatal accidents and to its withdrawal from service.
The first wide-bodied Boeing 747 began flying in 1969: the iconic wide-body jet was powered by large turbofan engines that developed more than 200 kN of thrust and could deliver a peak combined thrust of about 280 MW during take-off (Smil 2000c). By 2015 the most powerful jet engine, the GE 90–115B, was rated at 513 kN of thrust.
The only prime movers that can deliver more power per unit of weight than gas turbines are the rocket engines launching missiles and space vehicles.
6: Fossil-Fueled Civilization
Modern civilization depends on extracting prodigious energy stores, depleting finite fossil fuel deposits that cannot be replenished even on time scales orders of magnitude longer than the existence of our species.
Reliance on nuclear fission and the harnessing of renewable energies (adding wind- and photovoltaic-generated electricity to more than 130-year-old hydrogeneration, and turning to new ways of converting phytomass to fuels) have been increasing, but by 2015 fossil fuels still accounted for 86% of the world’s primary energy, just 4% less than a generation ago, in 1990
Unprecedented Power and Its Uses
In gross energy terms, the global supply of biofuels and fossil fuels was about the same in 1900 (both at roughly 22 EJ); by 1950 fossil fuels supplied nearly three times as much energy as wood, crop residues, and dung; and by the year 2000 the difference was nearly eightfold. But when adjusted for actually delivered, useful energy, the difference in the year 2000 was nearly 20-fold.
In foraging societies food was the only energizer; my estimate puts food and fodder at about 45% of all energy in the early Roman Empire (Smil 2010c). In preindustrial Europe food and fodder ranged from 20% to 60%, but by 1820 the mean was no more than about 30%; by 1900 it was less than 10% in the UK and Germany. By the 1960s fodder energy had declined to a negligible level and food had become no more than 3% and even less than 2% of all energy supply in the most affluent societies, whose consumption became dominated by industrial, transportation, and household uses of fuels and electricity (fig. 6.3). Per capita deliveries of electricity have risen by two orders of magnitude in high-income economies, by 2010 becoming around 7 MWh/year in Western Europe and about 13 MWh/year in the United States. Contrasts between energy flows controlled directly by individuals are no less impressive.
When in 1900 a Great Plains farmer held the reins of six large horses while plowing his wheat field, he controlled—with considerable physical exertion, perched on a steel seat, and often enveloped in dust—no more than 5 kW of animate power. A century later his great-grandson, sitting high above the ground in the air-conditioned comfort of his tractor cabin, controlled effortlessly more than 250 kW of diesel engine power.
In 1900 an engineer operating a coal-fired locomotive pulling a transcontinental train at close to 100 km/h commanded about 1 MW of steam power , the maximum performance permitted by manual stoking of coal (Bruce 1952; fig. 6.4). By the year 2000, pilots of a Boeing 747 retracing the transcontinental route 11 km aloft could choose an auto-mode for a large part of the journey as four gas turbines developed up to about 120 MW and the plane flew at 900 km/h
No gain enabled by this new power has been more fundamental than the substantial rise in global food production, which has made it possible to provide adequate nutrition to nearly 90% of the world’s population
Energy in Agriculture
Supplying nitrogen, the macronutrient always needed in the largest quantities per unit of cropped land, was the greatest challenge. Until the 1890s the only inorganic option was to import Chilean nitrates
None of these methods could supply fixed nitrogen on a mass scale, however, and the outlook for feeding the world changed fundamentally only in 1909 when Fritz Haber (1868–1934) invented a catalytic, high-pressure process to synthesize ammonia from its elements
Chickens are the most efficient converters of feed (about three units of concentrate feed for a unit of meat); the feed:meat ratio for pork is about 9, and the production of grain-fed beef is most demanding, requiring up to 25 units of feed for a unit of meat.
This inferior ratio is also the function of the meat:live weight ratio: for chicken it is as high as 0.65 and for pork it is 0.53, but for large beef animals it is as low as 0.38
But the energy loss in conversion to meat (and milk) has its nutritional rewards: the rising consumption of animal foods has brought high-protein diets to all rich nations (evident in taller statures) and has assured, on average, adequate nutrition even in most of the world’s largest poor populous countries. Most notably, the energy content of China’s average per capita diet is now, at about 3,000 kcal/day, about 10% ahead of the Japanese mean
Industrialization
Nor was mass consumption a real novelty. We tend to think of materialism as a consequence of industrialization, but in parts of Western Europe, especially in the Netherlands and France, it was a major social force already during the fifteenth and sixteenth centuries
Once energized by coal and steam power, traditional manufacturers could turn out larger volumes of good-quality products at lower prices. This achievement was a necessary precondition for mass consumption.
A radically new period of industrialization came when steam engines were eclipsed by electrification. Electricity is a superior form of energy, and not only in comparison with steam power. Only electricity combines instant and effortless access with the ability to serve very reliably every consuming sector except flying.
The availability of reliable and cheap electricity has transformed virtually every industrial activity. By far the most important effect on manufacturing was the widespread adoption of assembly lines
Their classic, and now outdated, rigid Fordian variety was based on a moving conveyor introduced in 1913. The modern, flexible Japanese kind relies on just-in-time delivery of parts and on workers capable of doing a number of different tasks. The system, introduced in Toyota factories, combined elements of American practices with indigenous approaches and original ideas (Fujimoto 1999). The Toyota production system (kaizen) rested on continuous product improvement and dedication to the best achievable continuous quality control. Again, the fundamental commonality of all of these actions is minimizing energy waste.
By far the most important innovation in nonferrous metallurgy was the development of aluminum smelting. The element was isolated in 1824, but an economical process for its large-scale production was devised only in 1866.
Transportation
As for the expense, just before World War I the cost of a transatlantic crossing averaged $75 (Dupont, Keeling, and Weiss 2012), or about $ 1,900 in 2015 monies. The return trip of nearly $4,000 in current dollars compares to about $1,000 for an average (undiscounted) London–New York flight.
Speed of a mile a minute (96 km/h) was first reached briefly on a scheduled English run in 1847; that was also the year of the greatest railway-building activity in the country, which laid a dense network of reliable links within just two generations (O’Brien 1983).
Certainly the most obvious car-generated impact has been the worldwide reordering of cities through the proliferation of freeways and parking spaces and the destruction of neighborhoods.
Car ownership has been an important part of embourgeoisement, and some affordable designs that enabled this new mass ownership enjoyed amazing longevity (Siuru 1989). The first was Ford’s Model T, whose price dropped as low as $265 in 1923 and whose production lasted 19 years (McCalley 1994). Other notable models were the Austin Seven, the Morris Minor, the Citroen 2CV, the Renault 4CV, the Fiat Topolino, and, the most popular of them all, Ferdinand Porsche’s Hitler-inspired Volkswagen
In terms of the aggregate production, size, and longevity (though with updated models), no car designed for the masses comes close to the one that Adolf Hitler decreed as the most suitable for his people (Nelson 1998; Patton 2004). In autumn 1933 Hitler set down the car’s specifications—top speed 100 km/h, 7 L/km, capable of conveying two adults and three children, with air cooling, and at a cost below 1,000 RM—and Ferdinand Porsche (1875–1951) had the car, rather ugly and looking, at Hitler’s insistence, like a beetle (Käfer), ready for production in 1938.
Information and Communication
The telephone, patented by Alexander Graham Bell in 1876 just hours ahead of Elisha Grey’s independent filing (Hounshell 1981), had an even faster acceptance in local and regional service (Mercer 2006). Reliable and cheap long-distance links were introduced rather slowly. The first trans-American link came only in 1915, and the transatlantic telephone cable was laid only in 1956. To be sure, radio-telephone links were available from the late 1920s, but they were neither cheap nor reliable. Great telephone monopolies provided affordable and reliable service, but they were not great innovators: the classic black rotary-dial telephone was introduced in the early 1920s and remained the only choice for the next four decades: the first electronic touch-tone phones appeared in the United States only in 1963.
Microchips have become the most ubiquitous complex artifacts of modern civilization: more than 200 billion of them are produced every year , and the devices can be found in products ranging from mundane household items and appliances (thermostats, ovens, furnaces, and in every electronic gadget) to the automated fabrication of complex assemblies, including the design and making of microprocessors themselves.
Economic Growth
To talk about energy and the economy is a tautology: every economic activity is fundamentally nothing but a conversion of one kind of energy to another, and monies are just a convenient (and often rather unrepresentative) proxy for valuing the energy flows.
Between 1900 and 2000 the use of all primary energy (after subtracting processing losses and nonfuel uses of fossil fuels) rose nearly eightfold, from 44 to 382 EJ, and the GWP increased more than 18 times, from about $2 trillion to nearly $37 trillion in constant 1990 monies (Smil 2010a; Maddison Project 2013), implying an elasticity of less than 0.5.
High correlations of the two variables can be found for a single country over time, but the elasticities differ: during the twentieth century, the Japanese GDP increased 52-fold and total energy use rose 50-fold (an elasticity very close to 1.0), while the multiples for the United States were, respectively, nearly 10-fold and 25-fold (an elasticity of less than 0.4), and for China nearly 13-fold and 20-fold (an elasticity of 0.6).
For example, Italy and South Korea have a very similar per capita GDP—adjusted for purchasing power, it was about $35,000 in 2014—but South Korea’s per capita energy use is nearly 90% higher than Italy’s. Conversely, Germany and Japan have a nearly identical annual energy consumption, about 170 GJ/capita, but in 2014 Germany’s GDP was nearly 25% higher
The primary energy (and electricity) intensity of global economic growth have been declining, but, because of the size of the world economy and the continuing population growth in Asia and Africa, the coming decades will repeat, though in a modified way, the past experience as large quantities of fuels and large additions of electricity-generating capacities will be required to energize economic growth in modernizing countries.
Consequences and Concerns
In the first category is an abundant food supply fostering indefensibly high food waste and contributing to unprecedented rates of overweight (a body mass index between 25 and 30) and obesity (body mass index >30). This trend toward heavier bodies is further reinforced by reduced energy expenditures, by more sedentary lifestyles resulting from mass replacement of muscle exertion by machines, and by the ubiquitous use of cars even for short trips that used to be made on foot.
By 2012, 69% of the U.S. population was overweight or obese, up from 33% during the 1950s (CDC 2015), a clear proof that those conditions have been acquired through the combination of overeating and reduced physical activity.
Urbanization
Rome of the first century CE housed more than half a million people.
Modern cities use fuels with much higher efficiency, but their high concentrations of housing, factories, and transport push their power density to 15 W/m2 in sprawling, warm-climate places and, in industrial cities in colder climates, up to 150 W/m2 of their area. However, both coals and crude oils supplying these needs are extracted with power densities ranging usually between 1,000 and 10,000 W/m2
This means that an industrial city needs to rely on a coalfield or oil field whose size is no more than one-seventh and as little as 1/1,000th of its built-up area, and on new powerful prime movers to transport fuels from their basically punctiform places of extraction to urban users.
All modern cities are creations of fossil energy flows converted with high power densities, but megacities make exceptionally high claims: a survey by Kennedy and co-workers (2015) concluded that in 2011 the world’s 27 megacities (with less than 7% of the global population) consumed 9% of all electricity and 10% of all gasoline.
And there is no doubt about the consequence of urbanization for energy consumption; living in cities requires substantial increases in the per capita provision of energy even in the absence of heavy industries or large ports: the fossil fuels and electricity required to sustain a person who moved to one of Asia’s new growing cities can be easily an order of magnitude higher than the meager amounts of biomass fuels used in the village of her birth to cook and (if need be) to heat a room.
Quality of Life
The real difference in typical direct per capita energy use among the richest and the poorest quarters of the mankind is thus closer to being 40-fold rather than “just” 20-fold. This enormous disparity is one of the few main reasons for the chronic gap in economic achievements and in the prevailing quality of life. In turn, these inequalities are a major source of persistent global political instability.
In the early stages of economic growth, these benefits are rather limited because fuels and electricity are overwhelmingly channeled into building up an industrial base. The slowly increasing acquisition of household and personal goods and better basic diets have been the first signs of improvement, starting in the cities and gradually diffusing to the countryside.
the low cost of new electric (air conditioning, microwave ovens, TVs) and electronic appliances and devices (above all mobile phones) means that in many Asian and in some African countries, families acquired them even before they owned other better household items.
But one of electricity’s most consequential social impacts has been to transform many chores of household work and hence to disproportionately benefit women. This change has been, even in the Western world, fairly recent.
As the standards of hygiene and social expectations rose with better education, women’s work in Western countries often got harder. No matter if it was washing, cooking, and cleaning in cramped English apartments (Spring-Rice 1939) or doing daily chores in American farmhouses, women’s work was still exceedingly hard during the 1930s. Electricity was the eventual liberator. Regardless of the availability of other energy forms, it was only the introduction of electricity that did away with exhausting and often dangerous labor
Political Implications
The Persian Gulf basin is an unparalleled singularity: it has 12 of the world’s 15 largest oil fields, and in 2015 it contained about 65% of the world’s reserves of liquid oil (BP 2015). These riches explain the lasting interest in the region’s stability. This desire is immensely complicated by the near chronic disarray of the area, which is made up of artificial states separated by arbitrary borders cutting across ancient ethnic group and containing complex religious enmities.
Weapons and Wars
As the basis for the first kind of comparison, it is useful to recall (as shown in chapter 4) that the kinetic energy of the two most common handheld weapons of the preindustrial era, arrows (shot from bows) and swords, was merely on the order of 101 J (mostly between 15 and 75 J), and that an arrow released from a heavy crossbow may hit a target with 100 J of kinetic energy. In contrast, bullets shot from the muzzles of muskets and rifles would have kinetic energies on the order of 103 J (10 to 100 times higher), while the shells fired from modern guns (including those mounted on tanks) rate at 106 J.
The Hiroshima bomb released 63 TJ of energy , about half of it as the blast and 35% as thermal radiation (Malik 1985). These two effects caused a large number of instant deaths, while ionizing radiation caused both instant and delayed casualties. The bomb exploded at 8:15 a.m. on August 7, 1945, about 580 m above ground; the temperature at the point of explosion was several million degrees Centigrade, compared to 5,000°C for conventional explosives. The fireball expanded to its maximum size of 250 m in one second, the highest blast velocity at the hypocenter was 440 m/s, and the maximum pressure reached was 3.5 kg/cm2 (Committee for the Compilation of Materials 1991). The Nagasaki bomb released about 92 TJ. These weapons appear minuscule compared to the most powerful thermonuclear bomb, tested by the USSR over Novaya Zemlya on October 30, 1961: the tsar bomba released 209 PJ of energy (Khalturin et al. 2005).
the Hiroshima bomb was equivalent to 15 kt TNT, the tsar bomba to 50 Mt TNT.
Yet another phenomenon that has greatly contributed to overall energy costs has been is the necessity to ramp up the mass production of military equipment in very short periods of time. The two world wars offer the best examples. In August 1914 Britain had only 154 military airplanes, but four years later the country’s aircraft factories were employing about 350,000 people and producing 30,000 airplanes a year.
When the United States declared war on Germany in April 1917 it had fewer than 300 second-rate planes, none able to carry machine guns or bombs, but three months later the U.S. Congress approved an unprecedented appropriation of $640 million (almost U.S. $12 billion in 2015 monies) to build 22,500 Liberty engines for new fighters
The 19 hijackers of 9/11 had no weapons other than a few box cutters, and the entire operation, including flight lessons, cost less than $500,000 (bin Laden 2004, 3)—while even the narrowest estimate of the monetary burden (New York City’s comptroller report, issued a year after the attack) put the city’s direct cost at $95 billion, including about $22 billion to replace the buildings and infrastructure and $17 billion in lost wages. A national perspective evaluating lost GDP, the decline of stock values, losses by the airline and tourist industries, higher insurance and shipping rates, and increased security and defense spending put the cost at more than $500 billion.
The verdict is simple: the United States does not need Iraqi oil, East Asia has been its largest buyer—so did the United States go into Iraq to secure Chinese oil supplies? Even the case seen by many as a clear-cut demonstration of energy-driven war is anything but! The conclusion is clear: broader strategic aims, whether well justified or misplaced, and not a quest for resources have led America into its post–World War II conflicts.
Environmental Changes
The consensus position is that, to avoid the worst consequences of global warming, the average temperature rise should be limited to less than 2°C, but this would require immediate and substantial curtailment of fossil fuel combustion and a rapid transition to noncarbon sources of energy—not an impossible but a highly unlikely development, given the dominance of fossil fuel in the global energy system and the enormous energy requirements of low-income societies:
7: Energy in World History
Energy Eras and Transitions
And the rise of the West owes a great deal to a powerful combination of two inanimate prime movers: to effective harnessing of wind and to the adoption of gunpowder, embodied by oceangoing sail ships equipped with heavy guns
The second simplification, the use of renewable versus nonrenewable energy sources, captures the basic dichotomy between the millennia dominated by animate prime movers and biomass fuels and the more recent past, heavily dependent on fossil fuels and electricity.
Long-Term Trends and Falling Costs
Automobiles and farm machines usually operate less than 500 hours a year, compared to over 5,000 hours for turbogenerators. Consequently, in terms of actual energy production, the global ratio between internal combustion engines and electricity generators is now about 2:1.
The efficiencies of prime movers are limited by fundamental thermodynamic considerations. Technical advances have been narrowing the gaps between best performances and theoretical maxima. The efficiencies of steam-driven machines rose from a fraction of a percent for Savery’s primitive engines to just over 40% for large turbogenerators of the early twenty-first century.
Only marginal improvements are now possible for turbogenerators, whether steam- or water-driven, but combined-cycle gas turbines can reach efficiencies of 60%. Similarly, the best combustors now perform close to theoretical limits. Both large power plant boilers and household natural gas furnaces may be up to 97% efficient.
In contrast, the everyday performances of internal combustion engines, the prime movers with the largest aggregate installed power, are still very low. Poorly maintained car engines often perform at less than a third of their rated maxima. Improvements in lighting efficiency have been even more impressive.
What Has Not Changed?
Classic account of business cycles in industrializing Western countries showed the unmistakable correlation between new energy sources and prime movers, on the one hand, and accelerated investment on the other
The first well-documented upswing (1787–1814) coincides with the spreading extraction of coal and with the initial introduction of stationary steam engines.
The second expansion wave (1843–1869) was clearly driven by the diffusion of mobile steam engines (railroads and steamships) and by advances in iron metallurgy.
The third upswing (1898–1924) was influenced by the rise of commercial electricity generation and by the rapid replacement of mechanical drives by electric motors in factory production.
Fascinating post-1945 research brought a great deal of confirmation about the existence of approximately 50-year pulsations in human affairs.
The economic consequences of the world’s prodigious energy use are also reflected in the roster of the world’s largest companies (Forbes 2015). In 2015, five of the top 20 nonfinancial multinational corporations were oil companies—EXXON, PetroChina, Royal Dutch Shell, Chevron, and Sinopec—and three were car and truck makers, Toyota, Volkswagen, and Daimler.
Another important ingredient of this affluence has been the use of energy to save time. These uses include much more than a widespread preference for more energy-intensive but faster private cars as opposed to the use of public transportation. Refrigeration (obviating daily food shopping), electric and gas ranges, microwave ovens and food processors (simplifying and accelerating the cooking or reheating of food), and central heating (eliminating the need for repeated kindling of fires and stocking up on fuels) have all been excellent time-saving techniques now universally adopted throughout the affluent world. In turn, the time gained by these energy investments is increasingly used for leisure trips and pastimes often requiring further considerable energy inputs.
On a more mundane level, tens of millions of people annually take intercontinental flights to generic beaches in order to acquire skin cancer faster; the shrinking cohort of classical music aficionados has more than 100 recordings of Vivaldi’s Quattro Stagioni to choose from; there are more than 500 varieties of breakfast cereals and more than 700 models of passenger cars. Such excessive diversity results in a considerable misallocation of energies, but there appears to be no end to it: electronic access to the global selection of consumer goods has already multiplied the choice available for Internet orders, and the customized production of many consumer items (using individualized adjustments of computer designs and additive manufacturing) would raise it to yet another level of excess. The same is true of speed: do we really need a piece of ephemeral junk made in China delivered within a few hours after an order was placed on a computer? And (coming soon) by a drone, no less!
By 2015 the richest 10% of humanity (living in 25 nations) claimed about 35% of the world’s energy.
Conversely, the poorest 5% of humanity (living in 15 African countries) consumes no more than 0.2% of the world’s primary commercial energy supply.
Imperatives of Energy Needs and Uses
The killing of large (meaty and fatty) mammals brought high net energy returns, while the hunting of smaller species was almost always a much less energy-rewarding pursuit than plant collecting.
Lipids were the most desirable nutrients commonly in short supply. Their high energy density provided the satisfactory feeling of satiety. These energy imperatives dictated collecting and hunting strategies and contributed to the emergence of social complexity.
The dominance of fossil fuels and electricity has created an unprecedented degree of technical and, by a gradual extension, also economic and social uniformity
The two most obviously worrisome consequences of the dependence on high energy flows is the restriction of choices (that is, the impossibility of abandoning existing practices without causing numerous massive dislocations) and degradation of the environment.
The Limits of Energy Explanations
Chronic conservatism (lack of imagination?) regarding the power of technical innovation, set against repeatedly exaggerated claims made on behalf of new energy sources.
Expert opinion of the day dismissed the possibility of gas lighting, steamships, incandescent light bulbs, telephones, gasoline engines, powered flight, alternating current, radio, rocket propulsion, nuclear energy communication satellites, and mass computing. This conservatism often persevered even after successful introduction of innovations.
Man perisheth. That may be, but let us struggle even though we perish; and if the nothing is to be our portion, let it not come to us as a just reward. (Senancour 1901 [1804], 2:187)